A laser emits a narrow beam of light, a unique characteristic that distinguishes it from other light sources and opens up a world of applications. This focused beam of light has a narrow width, unlike the diffused light emitted by traditional sources, making it ideal for precise and concentrated applications.
Laser beams exhibit exceptional properties, including low divergence and high intensity, which enable them to propagate over long distances without significant spreading. These properties make lasers essential tools in various fields, including manufacturing, medicine, research, and telecommunications.
Laser Beam Characteristics
Laser beams are renowned for their exceptionally narrow beam width, a characteristic that sets them apart from other light sources. This unique property enables lasers to focus their energy into a concentrated beam, resulting in remarkable applications in various fields.
The narrow beam width of lasers is attributed to their coherent nature. Unlike incoherent light sources, which emit light waves with random phases, lasers emit highly synchronized waves with a uniform phase. This coherence allows lasers to maintain a tight beam over long distances, minimizing divergence.
Factors Influencing Beam Width and Divergence
- Wavelength:Shorter wavelengths generally produce narrower beams due to the inverse relationship between wavelength and beam divergence.
- Optical Resonator Design:The design of the optical resonator, including the cavity length and mirror curvature, can influence beam width and divergence.
- Gain Medium:The gain medium’s properties, such as its length and homogeneity, can affect beam quality and divergence.
Laser Beam Propagation
Laser beam propagation refers to the manner in which a laser beam spreads and changes its characteristics as it travels through a medium. Understanding beam propagation is crucial for optimizing laser applications and designing optical systems.
As a laser beam propagates, its properties undergo gradual changes due to diffraction and other factors. Diffraction causes the beam to spread out, resulting in an increase in its divergence angle. This divergence is inversely proportional to the beam’s wavelength and directly proportional to the propagation distance.
Beam Quality and Propagation
Beam quality, which measures the beam’s spatial and temporal characteristics, is affected by propagation. Ideal laser beams have a low divergence angle, high beam quality, and maintain their characteristics over long distances. However, beam quality can degrade during propagation due to factors such as scattering, absorption, and turbulence.
Techniques for Controlling Beam Propagation
Various techniques can be employed to control beam propagation and maintain beam quality. These include:
- Beam shaping: Reshaping the beam’s cross-sectional profile to reduce divergence.
- Adaptive optics: Correcting distortions introduced by atmospheric turbulence or other factors.
li>Spatial filtering: Removing unwanted beam components that degrade beam quality.
Laser Beam Focusing: A Laser Emits A Narrow Beam Of Light
Laser beam focusing is the process of directing and concentrating the energy of a laser beam to a specific point or region. This technique is crucial for various applications, including laser cutting, welding, engraving, and medical procedures. By focusing the laser beam, it becomes more intense and can interact with the target material more effectively.
Factors Affecting Beam Focusing
The ability to focus a laser beam depends on several factors, including the wavelength of the laser, the optical properties of the focusing element, and the distance between the focusing element and the target. For optimal focusing, it is necessary to consider these factors and make appropriate adjustments.
Use of Lenses, Mirrors, and Other Optical Elements
Laser beam focusing can be achieved using various optical elements, such as lenses, mirrors, and diffractive optics. Lenses are commonly used to focus laser beams by converging or diverging the light rays, depending on the type of lens. Mirrors can be used to reflect and direct the laser beam, while diffractive optics can manipulate the wavefront of the laser beam to achieve specific focusing patterns.
Laser Beam Interactions
Laser beams interact with matter through various mechanisms, including absorption, reflection, scattering, and transmission. These interactions are highly dependent on the beam’s wavelength, power, and polarization, as well as the optical properties of the material.The absorption of laser energy by matter can lead to heating, melting, vaporization, and even ionization.
This interaction is utilized in applications such as laser cutting, welding, and surface modification. For instance, in laser cutting, a high-power laser beam is focused onto the material, causing it to melt and vaporize, resulting in a precise cut.Reflection occurs when a laser beam strikes a surface and bounces off without being absorbed.
The reflectivity of a material depends on its refractive index and the angle of incidence of the beam. Reflective coatings can be applied to surfaces to enhance or reduce reflectivity for specific applications.Scattering refers to the deflection of a laser beam due to irregularities or particles in the material.
This interaction can lead to beam divergence and loss of power. Scattering is often undesirable in applications where a focused beam is required, such as laser spectroscopy.Transmission occurs when a laser beam passes through a material without significant absorption or scattering.
The transmittance of a material depends on its thickness, absorption coefficient, and refractive index. Transparent materials, such as glass and water, have high transmittance, while opaque materials, such as metals and ceramics, have low transmittance.
Effects of Beam Parameters and Material Properties
The outcomes of laser-matter interactions are strongly influenced by beam parameters and material properties. The wavelength of the laser beam determines the depth of penetration and the type of interaction. For example, shorter wavelengths (e.g., UV) are more readily absorbed and can induce surface modifications, while longer wavelengths (e.g.,
IR) penetrate deeper and are more suitable for bulk heating.The power of the laser beam affects the intensity of the interaction and the resulting effects. Higher power lasers can generate higher temperatures and induce more pronounced changes in the material.The
polarization of the laser beam can also influence the interaction. For instance, in laser cutting, using a linearly polarized beam can produce a cleaner cut with reduced dross formation compared to a circularly polarized beam.Material properties such as thermal conductivity, specific heat, and melting point play a crucial role in determining the response to laser irradiation.
Materials with high thermal conductivity dissipate heat more rapidly, resulting in less localized heating effects. Materials with low melting points are more susceptible to melting and vaporization under laser irradiation.Understanding the interactions between laser beams and matter is essential for optimizing laser-based processes and applications.
By tailoring beam parameters and considering material properties, it is possible to achieve desired outcomes in laser cutting, welding, spectroscopy, and other applications.
Laser Beam Applications
Laser beams have revolutionized numerous industries and fields due to their unique properties and versatility. Their applications span a wide range, from manufacturing and medicine to research and telecommunications.
Manufacturing
- Laser Cutting:Precisely cutting materials such as metals, plastics, and ceramics with minimal heat-affected zones.
- Laser Engraving:Creating intricate designs and patterns on various surfaces for decorative or functional purposes.
- Laser Welding:Joining materials with high precision and strength, often in micro-scale applications.
Medicine
- Laser Surgery:Performing minimally invasive procedures with reduced bleeding and scarring, such as eye surgeries and tissue ablation.
- Laser Therapy:Treating various conditions, including pain management, wound healing, and skin rejuvenation.
- Laser Diagnostics:Using laser light to detect and analyze biological samples for medical research and diagnostics.
Research
- Laser Spectroscopy:Studying the interactions between laser light and matter to determine atomic and molecular properties.
- Laser Microscopy:Imaging microscopic structures with high resolution and depth penetration.
- Laser Ablation:Removing or analyzing specific materials from surfaces for geological or archaeological research.
Telecommunications, A laser emits a narrow beam of light
- Fiber Optic Communications:Transmitting data over long distances through optical fibers using laser beams as carriers.
- Laser Communication Systems:Establishing communication links between satellites, aircraft, and ground stations.
- Free Space Optics:Utilizing laser beams to transmit data through the atmosphere over short to medium distances.
Emerging Applications
Laser beams continue to find new applications in various fields:
- Additive Manufacturing:Using lasers to fuse or deposit materials for creating complex 3D objects.
- Quantum Computing:Exploiting laser beams to manipulate and control quantum systems for advanced computing.
- Laser-Induced Breakdown Spectroscopy:Identifying elemental compositions of materials through laser-induced plasma emission.
FAQ Explained
What is the advantage of a laser’s narrow beam?
The narrow beam of a laser allows for precise targeting, high intensity, and reduced divergence over long distances.
How is a laser beam focused?
Laser beams can be focused using lenses, mirrors, or other optical elements to concentrate the beam for specific applications.
What factors influence laser beam propagation?
Factors such as wavelength, beam diameter, and optical properties of the medium affect laser beam propagation and its characteristics.
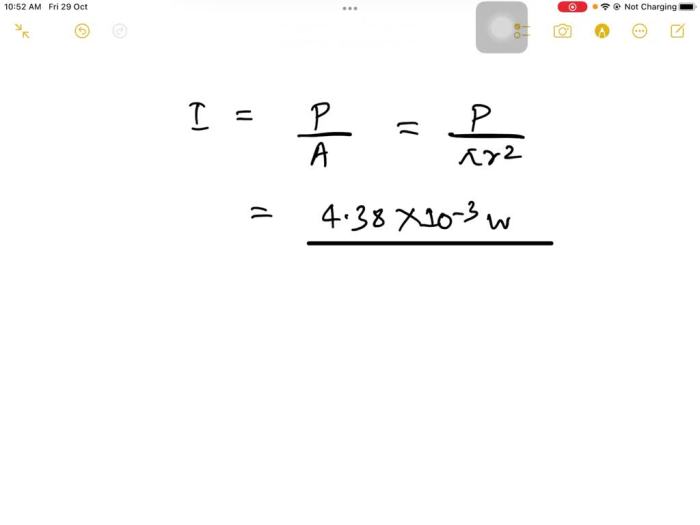